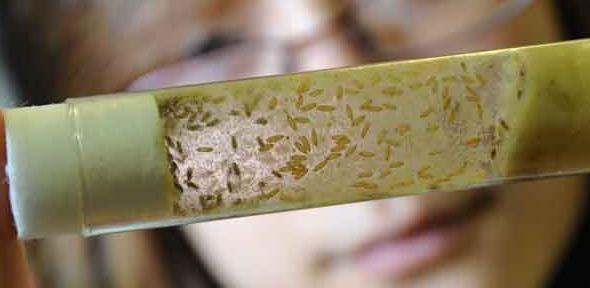
Since it first made its way into the laboratory a century ago, the fruit fly has helped transform biological research. Today, Cambridge is home to the greatest concentration of fly researchers in the world, and what they are discovering using this tiny...
“What's so striking is the enormous similarity at the genetic level between humans and flies”
Dr Damian Crowther
1908. It was the year that bank robbers Butch Cassidy and the Sundance Kid met their deaths in Bolivia, Baden-Powell set up the Boy Scout movement and the first Model T rolled off Henry Ford’s production line in Detroit, Michigan. Less well known, except among a close-knit community of scientists known affectionately as fly-pushers, is that 1908 also saw the introduction into the laboratory of a humble creature that over the past 100 years has become one of biology’s most powerful tools. Commonly called the fruit fly, Drosophila melanogaster is a small, brownish, two-winged fly with bright red eyes. Attracted to fermenting substances, most drinkers will recognise Drosophila as the creature that determinedly tries to drown itself in a glass of wine on a summer afternoon. Scientists were attracted to Drosophila for other reasons.
According to Professor Michael Ashburner of the Department of Genetics, who has worked with flies since the 1960s: “They are a good laboratory pet: very resistant to disease and easy to grow. They’re cheap, lay lots of eggs, each have a short generation time and, at 3mm long, they don’t take up much space.”
So economical are they that John Roote, who runs the Department of Genetics Fly Lab, reckons some 20 million Drosophila now reside in laboratories across the University. As well as being cheap and easy to raise, their chromosomes made them one of genetics most successful model species. “They have simple chromosomes – that was what first attracted scientists to them,” he says. “They have just four pairs of chromosomes and they posses what’s known as polytene chromosomes – giant chromosomes in the salivary glands that have a very reproducible banding pattern and are easy to observe,” he says.
Foundations of classical genetics
Drosophila and advances in genetics have gone hand in hand since 1910, when Thomas Hunt Morgan at Columbia University discovered a white-eyed mutation. By crossing white and red-eyed flies, Morgan’s team discovered the white eye was sex-linked – passed between generations of flies on the X chromosome and proof of the chromosome theory of heredity. Says Professor Ashburner: “Morgan accumulated a group of brilliant young researchers who, over the next ten years, laid the foundations of classical genetics. Before Morgan, the leading place for genetics worldwide was Cambridge, where researchers used a huge variety of organisms, from stud horses in Newmarket to chickens and plants, but their work lacked depth.”
During the decades that followed, discoveries made in Drosophila – and tools developed thanks to the fly – came thick and fast. Drosophila, whose genome was sequenced in 2000, became the test-bed for rapid sequencing so that this technology could be used to sequence the human genome.
The fly was also there for the start of genetic engineering. “Drosophila, although not alone, was certainly instrumental in introducing foreign genes into an organism. In my working life that's been their major contribution to science,” says Roote.
Thousands of postgraduate students have Drosophila to thank for their PhDs, and the fly netted several researchers – most notably Morgan in 1933, and Christiane Nüsslein-Volhard, Eric Wieschaus and Edward Lewis in 1995 – a Nobel Prize. Outside genetics, Drosophila has been equally influential, helping biologists such as Professor Pat Simpson in the Department of Zoology to understand how genes regulate development.
“When I started work 45 years ago, the fly had only really been used for Mendelian genetics and heredity, but several groups working on developmental biology thought Drosophila might be the one organism where we could try to understand how genes regulate animal development,” she says. What she and other developmental biologists discovered were the amazing similarities between fly and human: similarities that researchers at Cambridge are now exploiting to extraordinary effect.
Potential new treatments
In research that sounds more science fiction than science fact, Dr Damian Crowther in the Department of Genetics has created a fly model of Alzheimer’s disease. Thanks to a century's work into its genes and their role in development, Drosophila is now poised to help doctors understand what causes major human diseases and find potential new treatments.
“What’s so striking is the enormous similarity at the genetic level between humans and flies,” says Dr Crowther. “Flies have 14,000 genes, while humans have around 20,000, but if you look at disease-causing genes in humans, you find 70 per cent have an orthologue, or equivalent, in the fly. We’re using the brain of the fly as a system to model the human brain. Although the fly’s brain is much smaller than a human’s, the basic building blocks – the neurones – are very similar in structure.”
Over the past ten years, researchers have found that a small protein, known as the amyloid-β (Aβ) peptide, builds up in the brains of patients with Alzheimer’s, causing neurone death and dementia. Despite identifying Aβ as a molecular culprit, developing drugs to prevent its production have been dogged with unpleasant side-effects, meaning alternative drug targets must be found. Using genetic engineering techniques themselves partly developed in the fly, Dr Crowther has introduced a piece of human DNA that codes for the Aβ peptide into flies. After careful crossing, the flies produce the toxic peptide in their brains and begin to suffer problems that resemble Alzheimer’s in humans. Quite how you tell if a 3mm-long fruit fly has a poor memory or unsteady gait is mind-boggling, except to Dr Crowther.
In the same way Pavlov conditioned his dog to dribble on hearing a bell, flies can be trained to move towards different odours in a maze. They are trained to avoid one odour because it is associated with an electric shock, but flies with Alzheimer’s can't learn to avoid because they cannot remember. Training flies is labour intensive, so Dr Crowther prefers to examine how they walk to detect changes in their brains.
“We take a glass tube of flies and use two mirrors and a camera to reconstruct in 3D how they move up the tube,” she says. “Movement requires flies to be able to see and smell and walk – it’s a nice measure of overall brain activity – so we can use changes in the way they move to detect early signs of brain deterioration.”
Dr Crowther is using what he describes as “a test tube on legs” to get a greater understanding both of the disease process and how to interfere with it to stop Alzheimer’s progressing. “If we can interrupt that process in the fly, it’s very likely this will help us interrupt the process in a human brain.” His approach has already yielded promising leads. Working with the Department of Chemistry and Department of Medicine, Dr Crowther has found that iron seems to play a part in the disease process, and that binding the iron protects the flies from Alzheimer’s. “That gives us the ability to develop drugs to take the iron out of the human brain,” he says. Something to ponder, perhaps, next time you pick fruit flies out of your glass of wine.